By Aaron Foyer
VP, Orennia
In the 2008 James Bond film Quantum of Solace, the debonair spy and his accomplice, Camille Montes, are looking into the organization Quantum. A central member of the shadowy group is Dominic Green, ostensibly an environmentalist who is negotiating with the Bolivian government for a seemingly worthless piece of land in the country’s desert.
The movie’s climax takes place at Green’s luxury eco hotel, Perla de la Dundas, in the Bolivian desert. It’s here, in an arid hotel suspiciously powered by hydrogen fuel cells, where Bond and Montes discover the hidden value of the land: water.
The name’s O, H2O: Green’s ultimate scheme is to corner the country’s scarce freshwater resource and sell it to Bolivians at a marked-up price. Luckily, with the help of well-timed exploding fuel cells and some old-fashioned derring-do, Bond and Camille avert disaster and save the Bolivians from exploitation by evil resource barons.
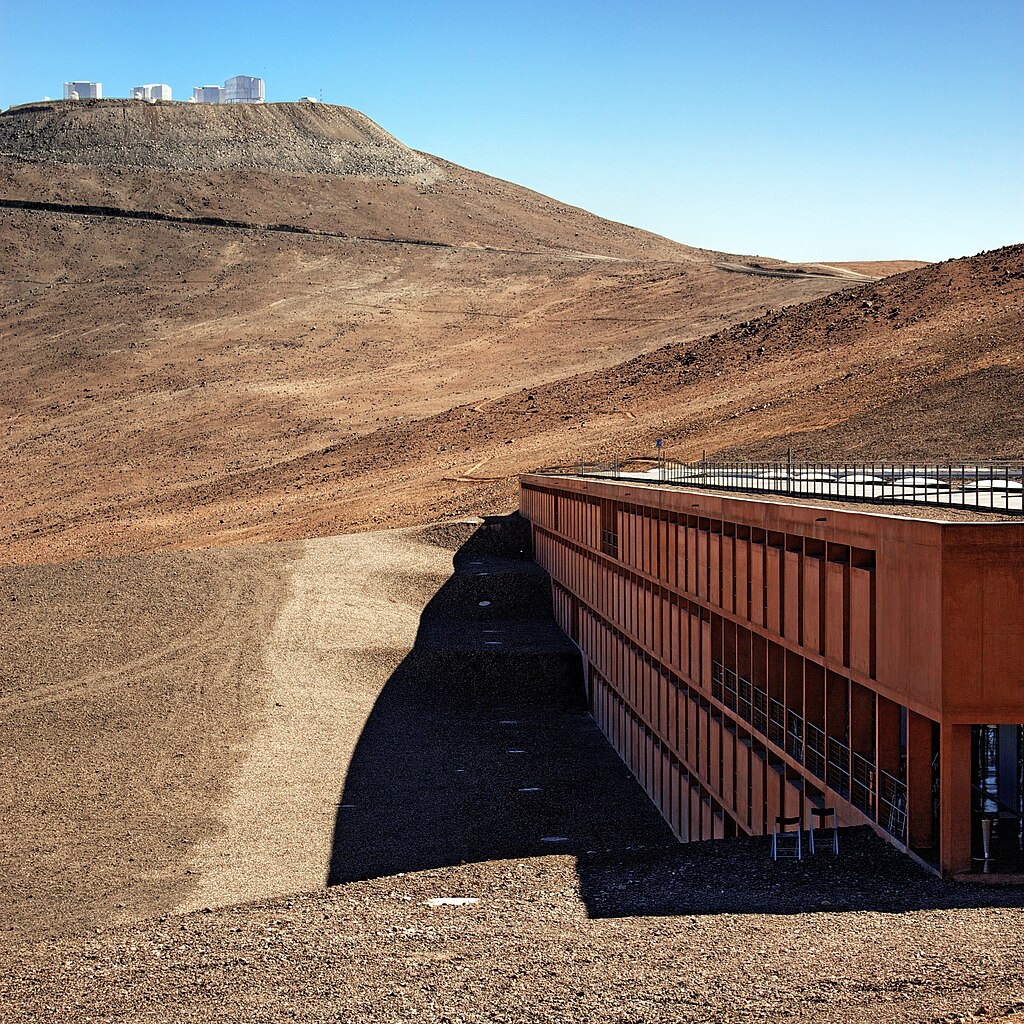
For many who watched the film, Green’s plan struck an unnerving chord: James Bond is often perfect escapism, but the water shortages felt too close to home. Between states contesting for river allocations and irrigation industries depleting aquifers, the liquid of life is increasingly in the headlines for all the wrong reasons. The energy sector is itself a huge consumer of water, and emerging low-carbon solutions could add new strain to water systems.
So, how critical is water to energy and could a shortage of available water hinder some emerging transition technologies?
Background
Water scarcity is a real issue in the US. Reservoirs in the Colorado River hit historic lows last year and nearly dead pooled, pushing Colorado, Arizona and Nevada to reach a historic water conservation agreement.
Freshwater abundance is no better below ground, where aquifers are regularly tapped by agriculture for irrigation systems faster than they’re being replenished. The US Geological Survey found pumping has lowered the water levels in a deep basalt reservoir in Oregon by more than 100 feet since 1965.
On energy: Given irrigation uses more freshwater than a Gwyneth Paltrow skincare routine, it may come as a surprise that the power sector, not agriculture, is the single largest water user in the US.
Estimated US Water Flow Diagram, 2011
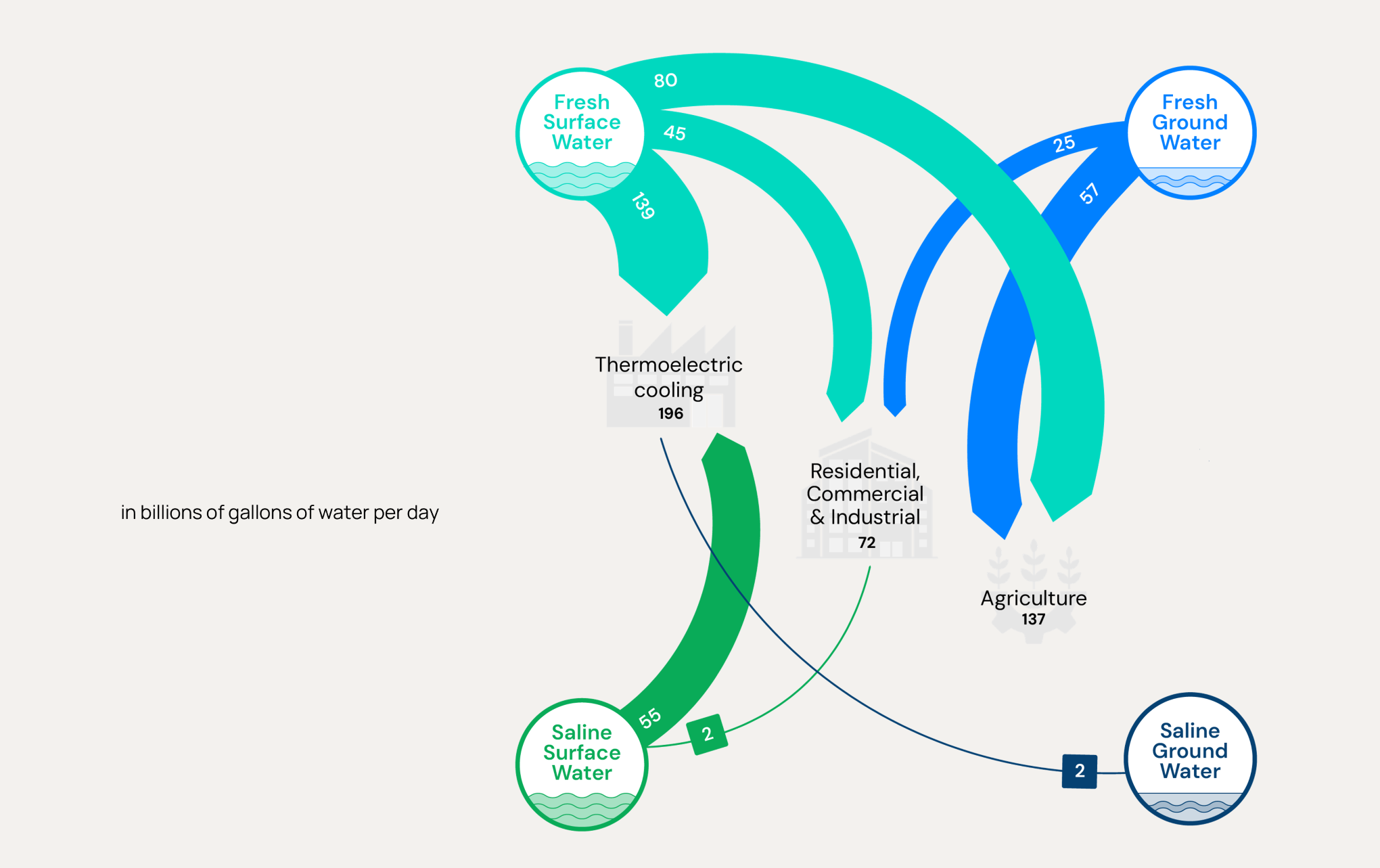
In a slightly dated study of water use across the country from 2011, the cooling of thermoelectric power plants accounted for more than half of all American water use. Nearly all came from surface sources—rivers, lakes and seawater. While much of that is recycled and modern power plants are more water efficient, it nonetheless highlights that the sheer scale of the energy sector can impact water at a country level.
With limited supply, there are worries that emerging technologies like green hydrogen, data centers and biofuels could either intensify water shortages or have water access limit their growth.
Water use in energy systems is complex
To better understand how energy impacts water systems, it’s important to clarify a key concept: not all water use is permanent. The US Energy Information Administration (EIA) distinguishes between water withdrawal and water consumption for power plants.
- With withdrawal, water is returned to the original water body after it’s been used, often due to cooling towers that condense water vapor.
- By contrast, consumption means water is not returned to the original source. This could be from evaporation, being incorporated into products like hydrogen or waste.
For the cooling of thermal plants, one of the single largest users of water in the US, 98% of water use is withdrawals, meaning water is borrowed and returned. It’s no coincidence that nearly every thermal power plant in the US is located on a river, lake or ocean for access to surface water.
Average Water Intensity of Thermoelectric Cooling by Plant Type
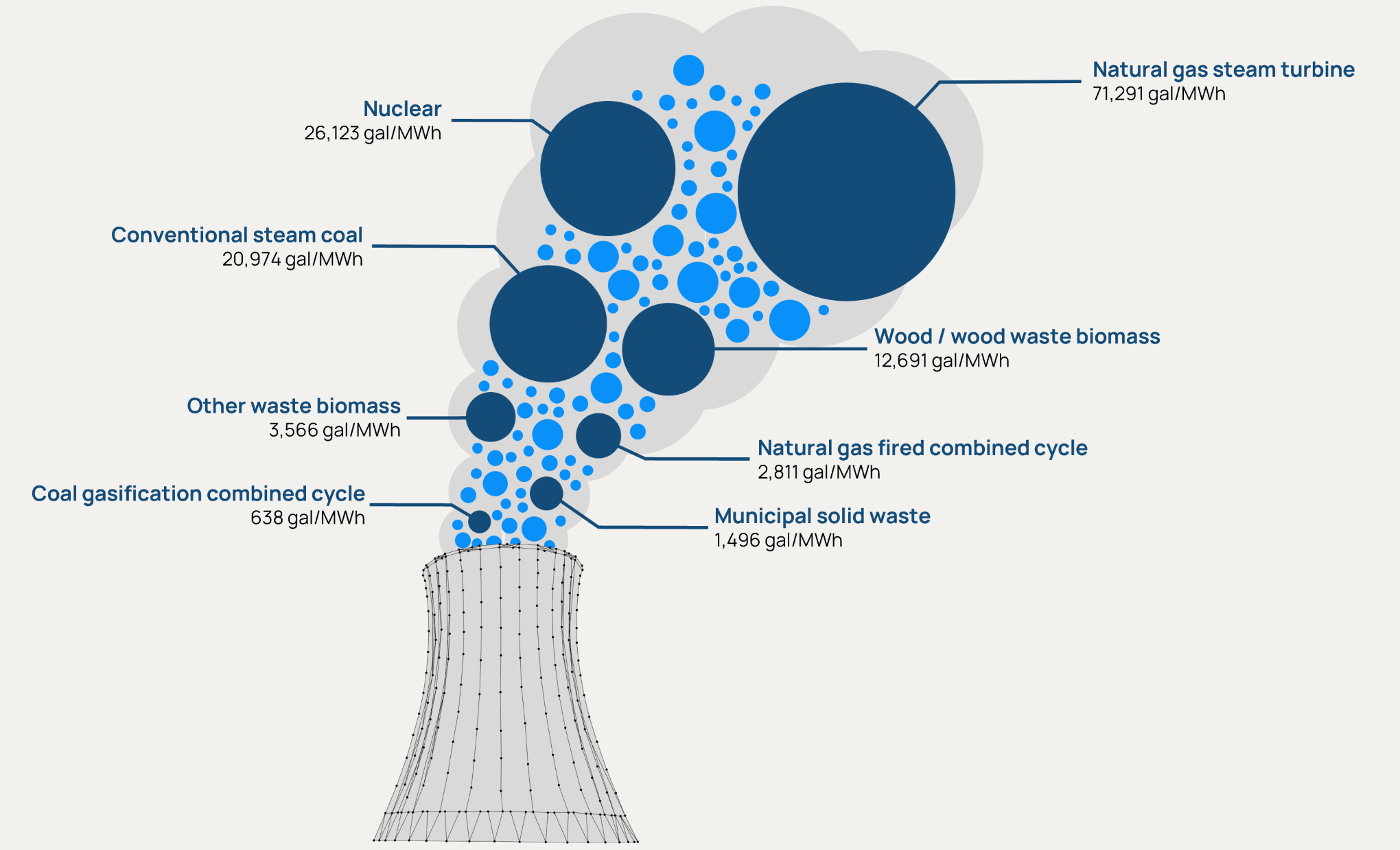
But while many thermal plants use and return water, that’s not always the case.
Sequestration and evaporation: Water is often injected underground but not returned, including by the natural gas industry, which uses extensive volumes for hydraulic fracturing. While some is recovered and recycled, the American Geoscience Institute estimates 90% of water used by the industry is disposed of underground. That’s effectively taking water out of the water cycle and sequestering it underground.
Evaporation is another issue. Like a Bubly warming up on the banks of Lake Mead, summer heat takes a toll on water at hydro dams. It’s believed Lake Mead, the reservoir created by the Hoover Dam, loses six vertical feet of water, or 6% of its total volume, due to evaporation every year. While this evaporation stays within the water cycle, it’s still often lost to the downstream river. In the case of Lake Mead, the evaporation is from the Colorado River, a water system that services 40 million people across seven states and irrigates 15% of American agriculture.
What will the energy transition bring?
As we shift from looking at existing energy systems to future ones, water will remain an issue. This is especially true for biofuels, green hydrogen and data centers.
The biomass dilemma: You could be forgiven for thinking, after just learning 6% of Lake Mead is lost to evaporation every year, that hydropower is the largest consumer of water amongst the different energy types. Not so. That title is held, by an order of magnitude, by biomass.
Water Consumption Over the Lifecycle of Generation Types
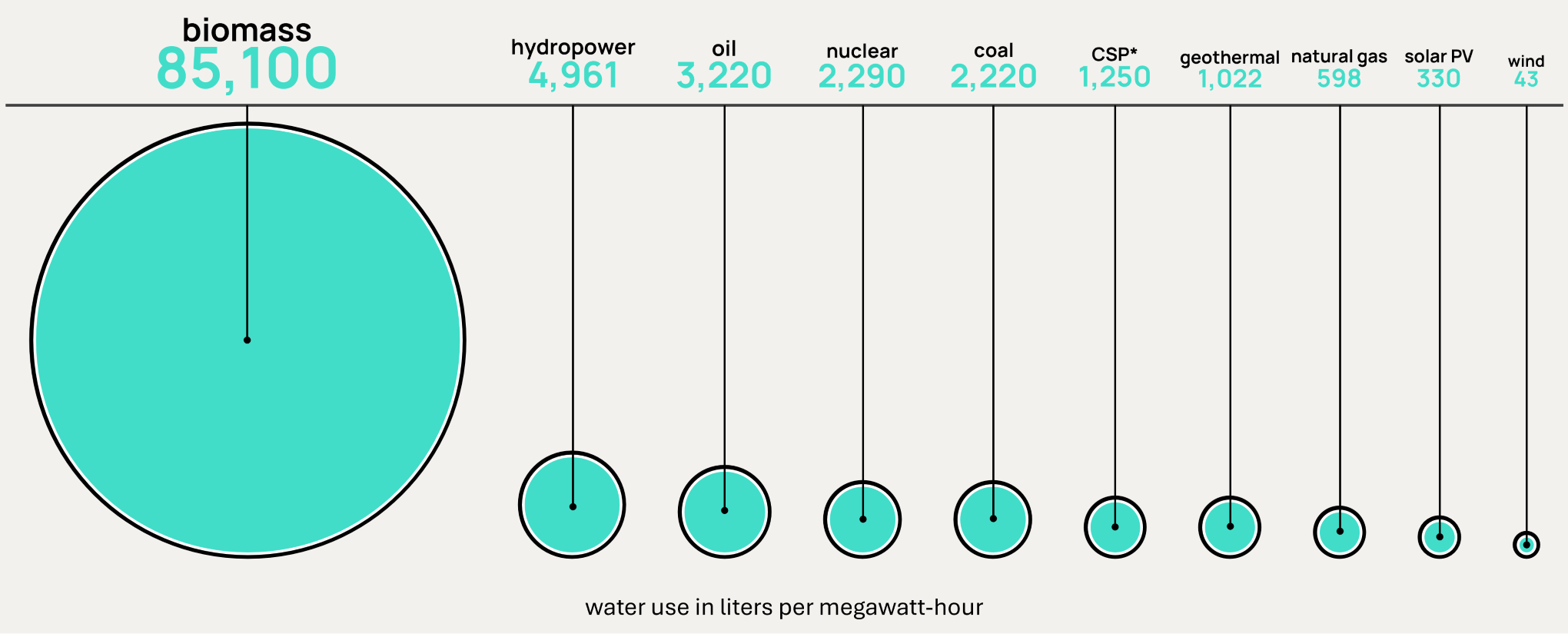
As anyone who has ever tried to keep ferns alive at home will tell you, plants consume a lot of water. A recent peer-reviewed study published in the Renewable and Sustainable Energy Reviews journal looked at how much water different energy generation types use over their entire lifecycle.
When including water for generating the fuel (which includes things like hydraulic fracturing, operations and even growing trees), nothing holds a candle to biomass. To be clear, water use is not currently an issue as the majority used to make electricity is waste wood grown with natural rainfall. But should there be an intensive focus on growing (pun intended) industries like bioenergy with carbon capture and storage (BECCS) projects, water will eventually need to be taken out of the water cycle for electricity generation and carbon removal. Far from fantasy, there are already large BECCS projects moving forward, including a $2.5 billion project recently approved in the UK that would capture and store 4 million tons of carbon emissions every year.
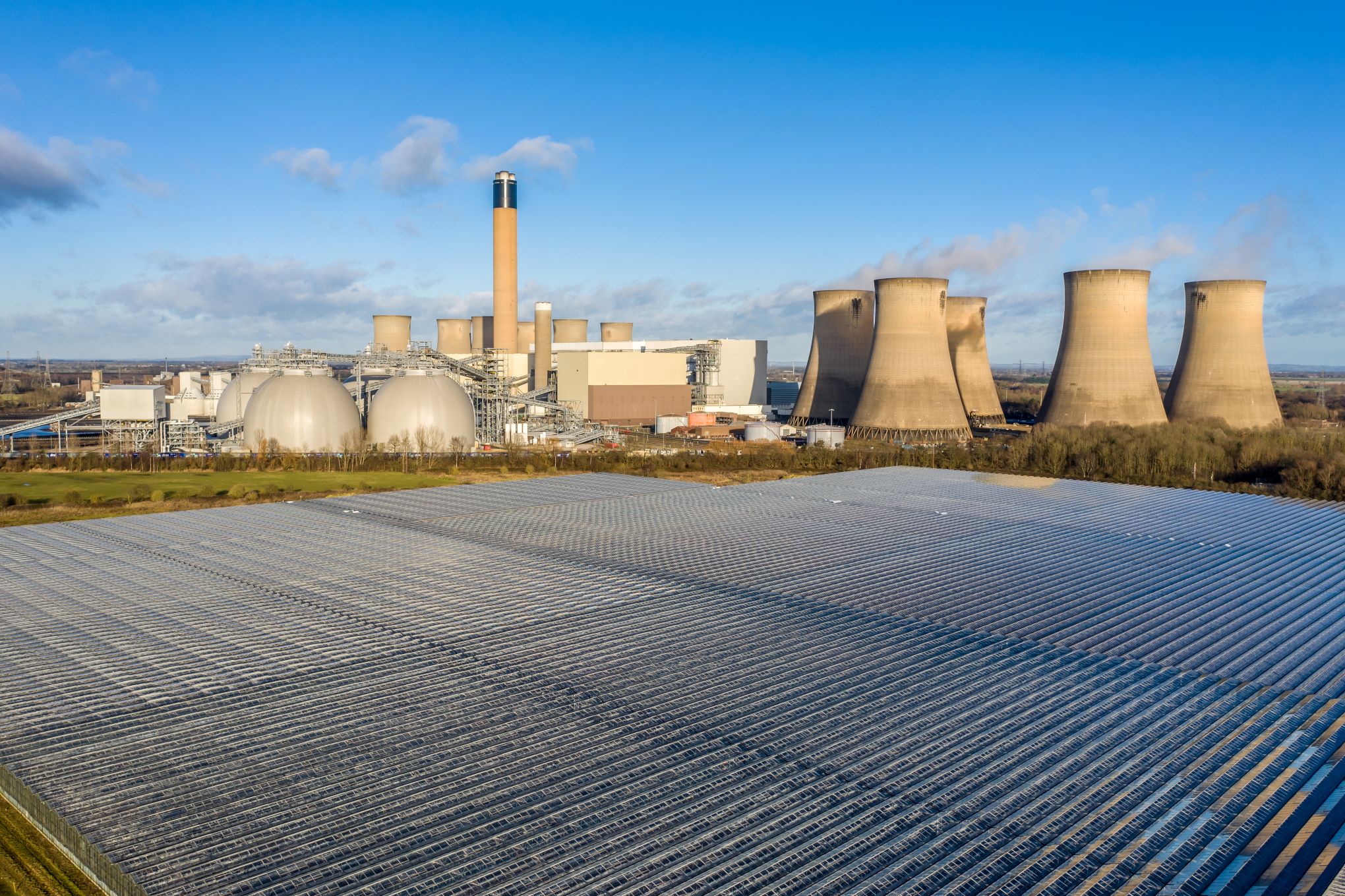
Other biofuels are similarly challenged. Ethanol made using irrigated crops is by far the most water intensive transportation fuel around.
Green hydrogen: The process of taking a water molecule and then splitting it with electricity obviously removes water from the system. When used, hydrogen often reconstitutes back into water, so the total water gains and loses might be neutral in the grand scheme. But that doesn’t stop water from being a significant barrier to locating electrolyzers.
On top of any water used for generating electricity and operations, each kilogram of green hydrogen requires nine kilograms (liters) of water for splitting. And not just any water: electrolyzers need ultra-purified water. Securing access to enough water in the right locations looks like a challenge for many developers. Highlighting the uphill battle the industry faces, a study last year conducted by Water Management Solutions found that the full development of the hydrogen sector in Alberta was “likely to both cause water supply challenges and be limited by water availability.”
US green hydrogen projects already face the challenge of addressing the three pillars for tax credits and finding a geographic unicorn at the center of the solar resource-wind resource-hydrogen offtaker Venn Diagram. Securing water rights represents another hurdle to overcome.
And, of course, data centers: To handle the heat generated from all those roasted radish recipe searchers, servers require cooling. There are several ways to manage heat in data centers, the two most common being air cooling and liquid cooling. While air cooling has historically been the stalwart technology of heat management, the future looks to be liquid.
To continuously improve computer performance, microchips have seen an increase in transistor density. As a byproduct of this, the newest chips give off too much heat for air cooling to handle, so future data centers are increasingly turning to liquid cooling for the task. Liquid cooling also helps reduce the noise created by air cooling, which has been generating complaints from nearby communities.
Select water-intensive energy facilities in the US
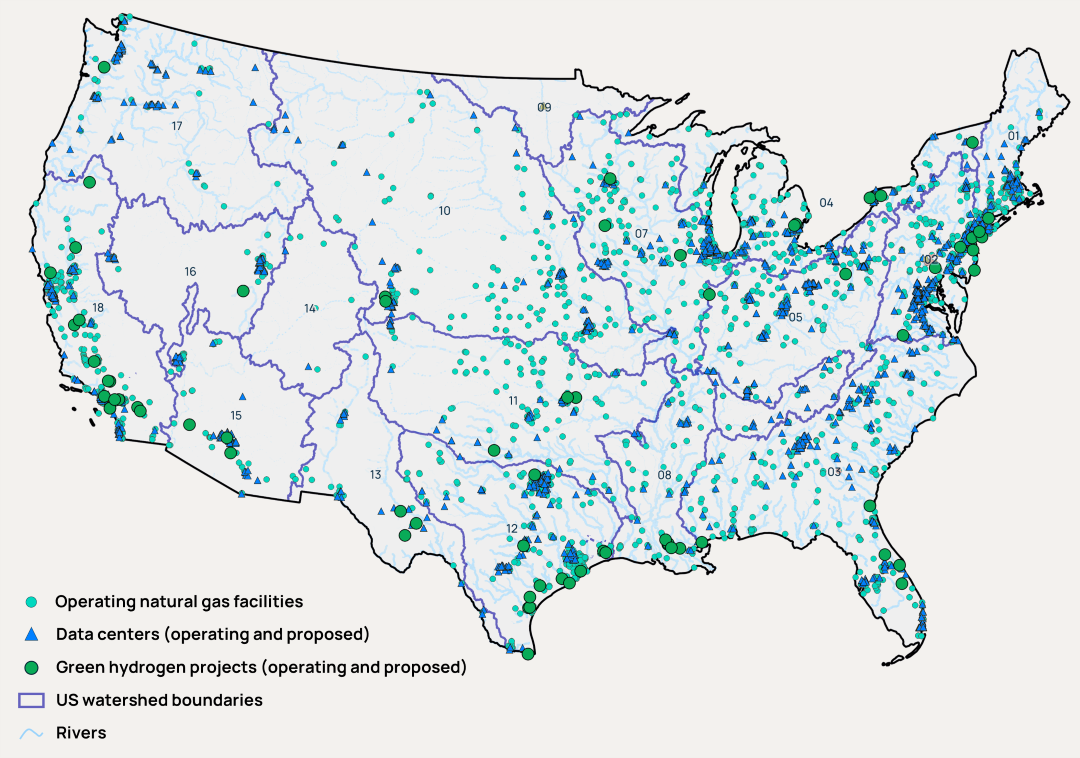
Your average data center consumes 1.8 liters per kilowatt-hour of electricity use. At the facility scale, that means a typical 60-megawatt hyperscaler data center consumes about an Olympic swimming pool worth of water every day. Being able to source enough water for cooling is an important consideration for developers, and something that can get Big Tech companies in trouble with the public.
Zooming out
American Founding Father Benjamin Franklin put it best, capturing how we take the value of water for granted. “When the well is dry, we know the worth of water,” Franklin said.
While the energy sector has historically been one of the largest users of water in the US, the uses were mostly withdrawals, returning water back to where it came from. The future energy sector could be very different, with biofuels, green hydrogen and other energy users consuming already scarce water.
Then there is the problem of securing enough water, with understanding water rights being a topic worthy of its own deep dive. In eastern states where water is more plentiful, rights are riparian, meaning they’re tied to ownership along waterways and more collectively managed. This is in contrast to western states, where water is scarcer, and principles of prior appropriation are used instead.
Bottom line: Planning future energy facilities for water reasons alone is no small feat. The reason Dominic Green’s plan in Quantum of Solace was so unnerving was that it rang true to real world challenge. Water is a scarce resource, and we all know it.
Maybe Green was an environmentalist after all, just a green hydrogen developer trying to secure some water rights.
Sparked thoughts
- An obstacle for biofuels? It’s obvious when you think about it that biofuels use a lot of water, but it’s not common knowledge. Scaling biofuels to meaningfully displace fossil fuels may spark water availability constraints.
- The real green hydrogen killer? Having water rights on top of the three pillars of green hydrogen development is tough. The additionality, deliverability and temporal matching requirements for tax credits already seem like an unfair burden for the burgeoning industry.
- Thermal retirements = data center opportunity? With the number of coal, gas and nuclear plants being retired, the volume of water being withdrawn for thermoelectric cooling will go down. Data centers, which withdraw rather than consume most of their water, could benefit from freed volumes.
Aaron Foyer is Vice President, Research and Analytics at Orennia. Prior to Orennia, he leveraged his technical background in management consulting and finance roles. He has experience across the energy landscape including clean hydrogen, renewables, biofuels, oil and gas, petrochemicals and carbon capture.